Our goal is to combine the fields of analytical chemistry, chemical biology, and material science to solve important problems in biology and medicine. To this end, we are focused on the following research areas:
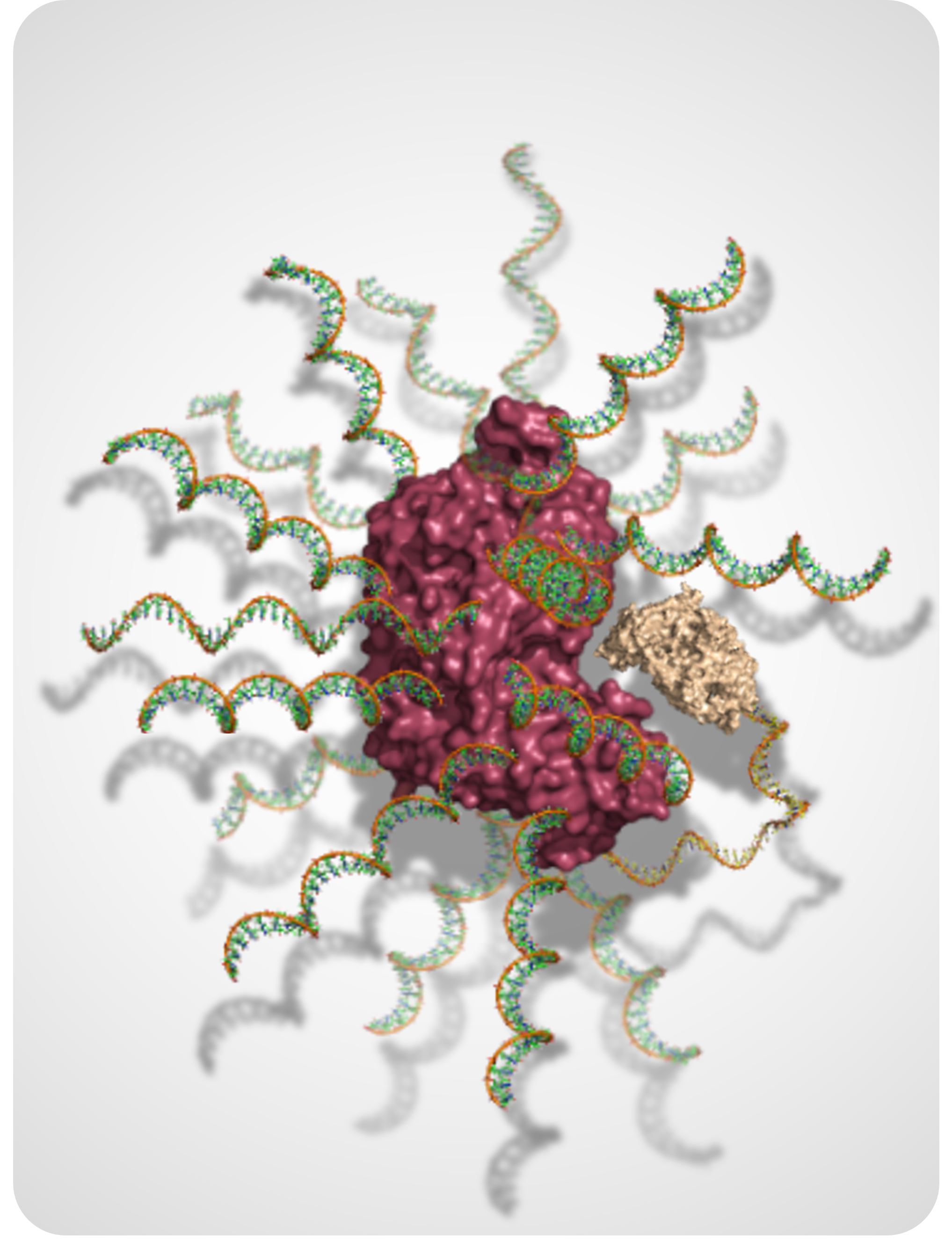
Protein-DNA Nanostructures for Biosensing
Proteins and DNA are the foundation of some of the most sensitive detection tools, like PCR, which was widely used to detect SARS-CoV-2 during the COVID-19 pandemic. We are developing nanostructures that harness the strengths of proteins and DNA while also overcoming their weaknesses, such as stability, speed, and the conditions they need to work in. For example, we are developing nanostructures that can enter cells—a feat not possible with proteins and DNA alone—to detect disease-related molecules directly inside living cells. This structures have the potential to significantly enhance our understanding of diseases like cancer by enabling the detection of specific molecules at the single-cell level. In addition to advancing basic biological research, these nanostructures offer practical applications, such as early disease diagnosis, the isolation of rare cells like circulating tumor cells based on their intracellular makeup, and improved precision in image-guided surgeries. Furthermore, we are developing biosensors for point-of-care testing, which could make disease detection faster and more accessible.
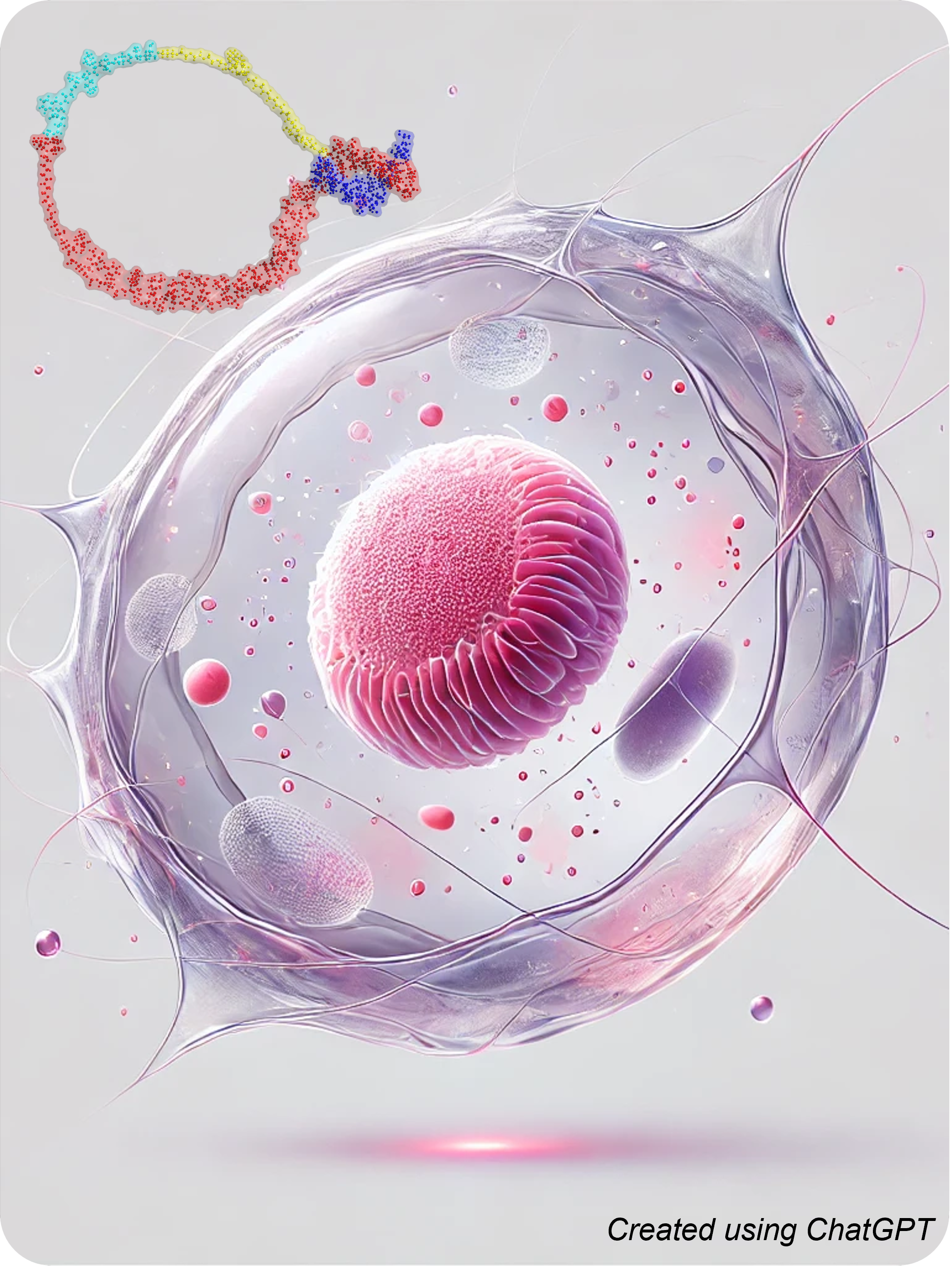
Chemical Activity as a Fingerprint of Cellular Identity
Over the past ~150 years, a central challenge in biology has been to catalog cells into distinct types to understand their functions in multicellular organisms and distinguish between healthy and diseased states. We are developing new technology that shifts the paradigm of cellular classification from focusing on molecular abundance to molecular activity. The rationale is simple: a cell's function is determined by the activity of its molecules, not just their presence. We hypothesize that an activity-based classification will more accurately reflect cellular functionality. This approach addresses the limitations of traditional transcriptomic and proteomic methods, both of which fail to differentiate between active and inactive molecular forms. This new method could reveal new cellular states and reshape our understanding of cellular identity. Moreover, it could lead to advances in early detection of cancer and other diseases, by enabling precise cell identification based on activity levels. Chemical activity of cells could also be used to assess clinical outcomes for tumor aggressiveness and metastasis.
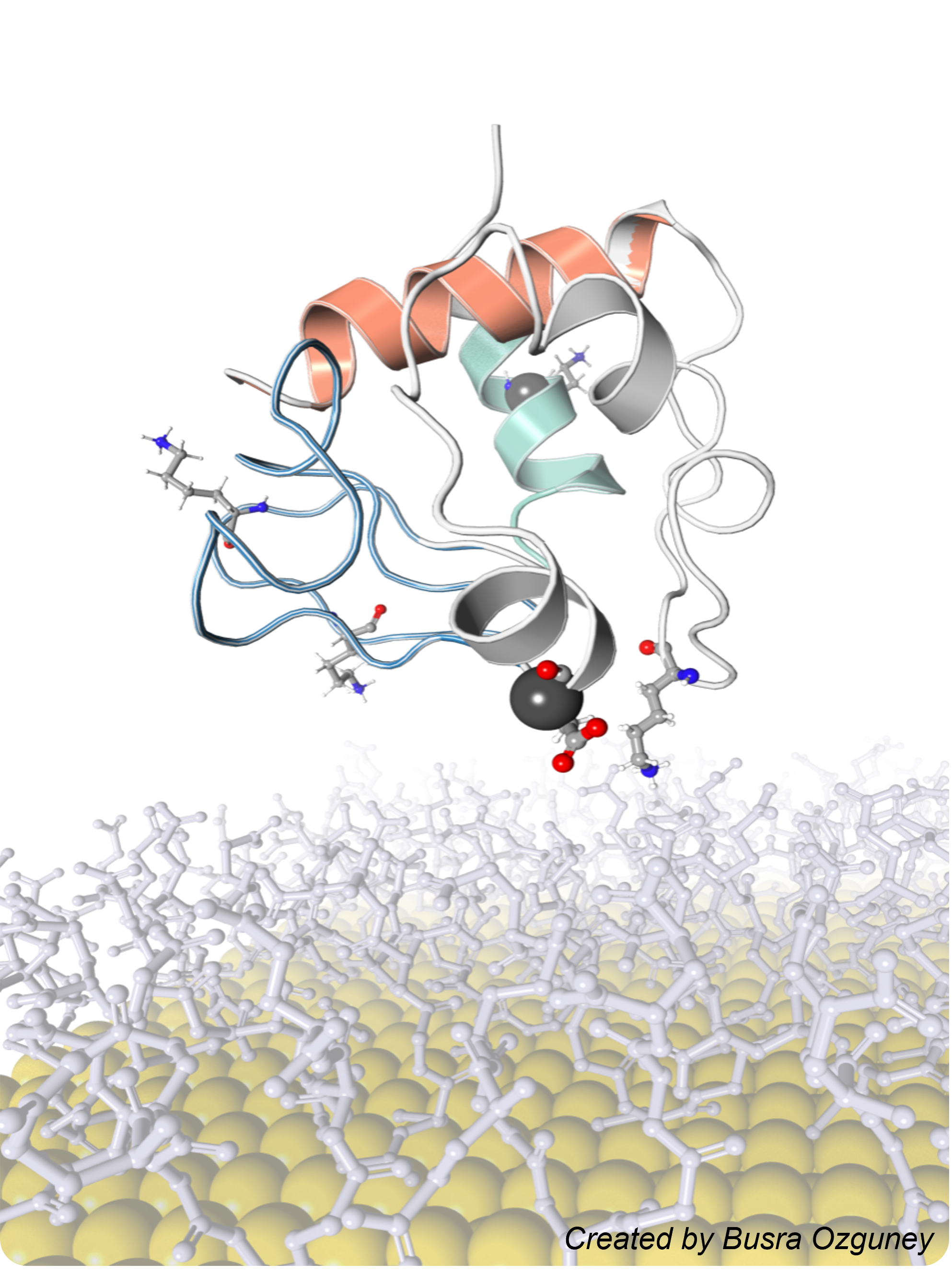
Designer Nanomaterials for Enhancing Enzyme Activity
Enzymes are key players in a variety of technological advancements, contributing to a global market valued at approximately $15 billion. Their remarkable ability to speed up chemical reactions with high specificity makes them indispensable in industries like pharmaceuticals, food production, industrial processes, and environmental solutions. Consequently, precise control over enzyme activity can greatly enhance these applications. Traditionally, enzymes have been immobilized on nanostructured materials to improve their stability and reusability. However, this approach has often resulted in significant losses in enzyme efficiency, with activity reductions of over 90% in some cases. Our research focuses on developing nanomaterials that not only bind to enzymes but also significantly boost their activity—by more than 10-fold. Furthermore, we are designing nanomaterials capable of dynamically tuning enzyme activity in response to user-defined cues, offering exciting new possibilities for precise and adaptable control of enzyme functions across various applications.
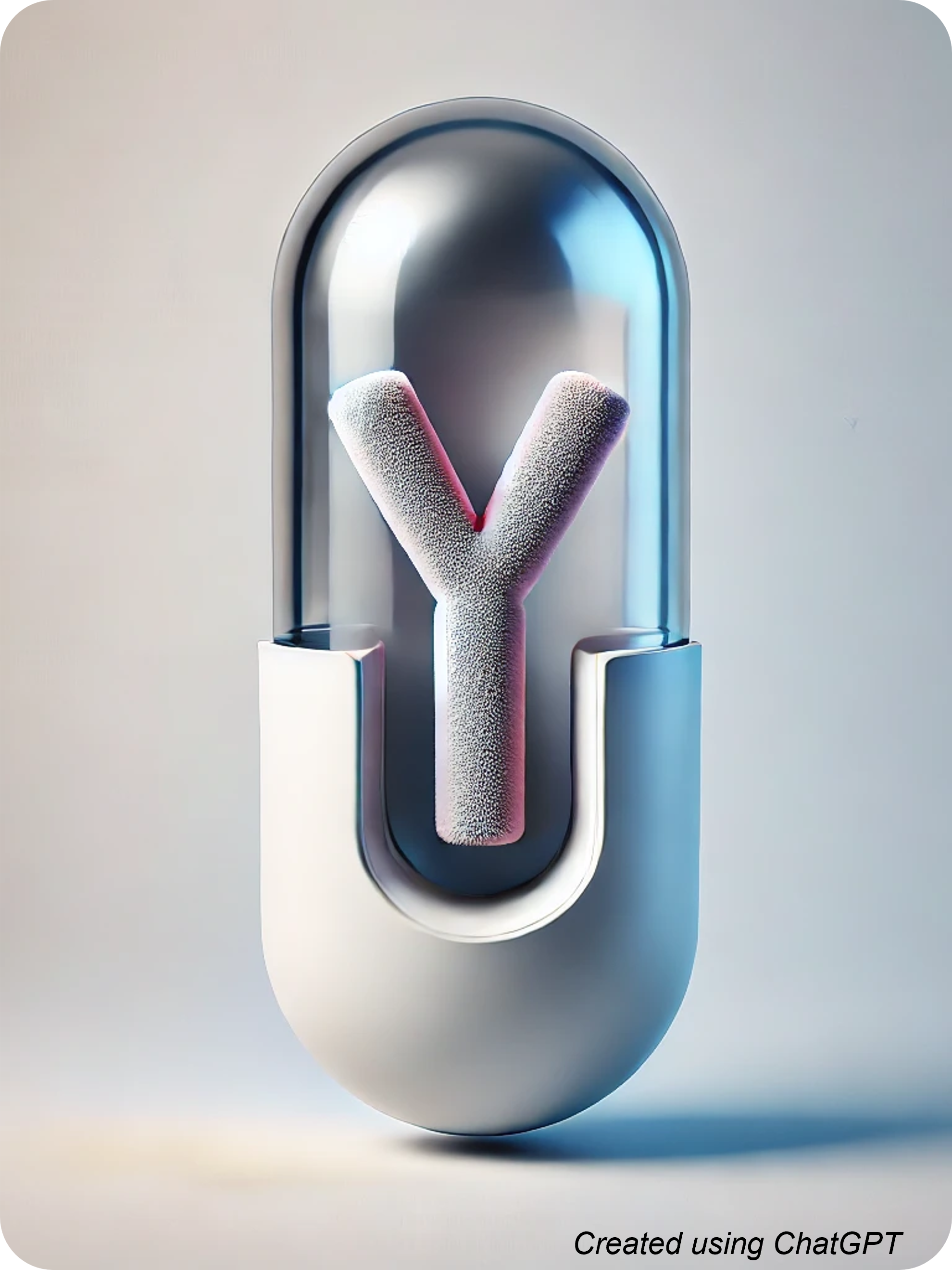
Intracellular Protein Delivery
Protein-based therapeutics have revolutionized biology and medicine, with a global market valued at over $100 billion. These therapeutics are at the forefront of biotechnological innovation, with more than 100 FDA-approved protein-based drugs and hundreds more in clinical trials. In 2023, six of the top ten best-selling drugs were protein-based, underscoring their growing competition with small molecule drugs. However, a major challenge persists: proteins cannot efficiently enter cells, limiting their ability to target intracellular components. As a result, there are few FDA-approved protein therapeutics for intracellular targets and none for antibodies. Our research focuses on developing methods to deliver proteins and antibodies into cells, a potential breakthrough that could enable treatment of diseases by targeting previously "undruggable" intracellular proteins.